Rational design, synthesis, and characterization of polymers and porous materials for energy-efficient separations
Core Areas of Expertise
Materials Design

The Smith Lab currently focuses on the rational design, synthesis, and characterization of polymers, metal-organic frameworks (MOF), and hybrid materials such as polymer-MOF mixed-matrix membranes (MMM). Our projects are targeted towards improving separation performance and stability as well as understanding the appropriate structure-property effects leading to improved separation in order to rationally design future materials.
- Creating sub-nanometer features in amorphous polymers:
- The ability to control the size distribution of free volume elements in a polymer membrane is of great interest for gas separations. In this study, functionalized polymer membranes will be post-synthetically treated to simultaneously remove functional groups to create free volume elements. Through this technique, specifically-sized free volume elements can be generated allowing for precise control of the membrane diffusivity selectivity. The ability to tune the pore-volume distribution has the potential to improve purity of size-based separations such CO2 capture from flue gas and oxygen-enriched air.
- Elucidating the role of fluorine on molecular interactions:
- Perfluorinated polymer membranes (all C-H bonds replaced with C-F bonds) have recently become a material of interest for certain gas separations such as He/H2 and N2/CH4. Additionally, these materials have shown remarkable stability over long time periods as well as in the presence of aggressive gas mixtures. In general, fluorine is known for its anomalous thermodynamic mixing interactions with other molecules, and gas solubility in perfluorinated membranes is no exception. This project aims to understand the role of fluorine on gas solubility and how those anomalous interactions result in improved separation performance.
- Expanding polymer chemistry toolsets for separations:
- In the framework of this project, we aim so synthesize brand new polymer structures making use of fine chemistry methods such as Catalytic Arene-Norbornene Annulation (CANAL) Polymerization and Ring Opening Metathesis Polymerization (ROMP). Through the use of novel chemistry methods, we hope to uncover new methods of analyzing structure-property effects related to gas diffusion and solubility to produce CO2-selective polymers that can efficiently separate this gas from other light gases such as CH4, N2 and H2 for sustainable energy applications.
- Precisely designing and characterizing the polymer-MOF interface in hybrid materials:
Crystal structures of MOFs containing coordinatively unsaturated metal sites. - The incorporation of mostly-inorganic MOF materials in organic polymer membranes results in compatibility issues at the materials interface. This interfacial challenge will primarily be addressed through modification of the MOF interface with organic functional groups or polymer chains to allow for better physical or chemical adhesion to the polymer matrix. This technique of MOF modification will be applied to a variety of polymer-MOF systems with the goal of developing a general method towards addressing interfacial compatibility in hybrid materials.
- Creating structured crystalline films:
- Metal-organic frameworks have attracted great attention in the realm of gas storage and separations, offering exceptional energy efficiency in many relevant industrial separations. In addition to high thermal and chemical stability, growing MOFs as thin films enables the possibility of a continuous, defect-free layer which selectively permits certain molecules. MOF films are not easily processable, making it difficult to form continuous films and preventing accurate determination of the true separation performance of pure MOF materials. Fabrication of continuous films provide a valuable method for determining transport properties for pure MOF films and for assessing the ability to scale up MOF materials for industrial membrane-based applications.
Application Targets
Our materials research is tailored towards addressing large-scale and impactful issues across a variety of gas and liquid phase separations.
- Carbon capture:
General schematic for CO2 capture from flue gas using a MOF crystalline film. - A major player in climate change is the release of greenhouse gases into the atmosphere, specifically of CO2 in the flue gases of power plants. Membrane materials have the potential to separate CO2 from flue gas streams for future sequestration.
- Energy efficiency:
- Currently, the primary continuous method for industrial gas separations is cryogenic distillation, an energy-intensive method due to the low temperature required to condense gases. If certain performance metrics can be met, membrane-based separations could replace cryogenic distillation as an energy-efficient alternative for a large amount of separations.
- Water purification:
- Clean water is expected to be a pressing issue in the near future. Research into new membrane materials for reverse osmosis and filtration of contaminants such as boron is essential towards development of a low-cost process for clean water.
- Functional composites:
- Functional composites are a class of hybrid materials that combine the desirable properties of several materials to produce a new material possessing intrinsic functional properties (e.g. separation, conductivity, etc.). Research into functional composites could reveal new hybrid materials that could improve on the properties/performance of current materials.
Advanced Characterization
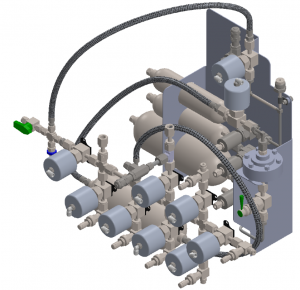
The Smith Lab has the capability to fully characterize novel polymer and MOF materials using various in-house equipment and shared facilities on the MIT campus, including thermogravimetric analysis, differential scanning calorimetry, nuclear magnetic resonance, Fourier transform infrared spectroscopy, x-ray diffraction, etc.
- Automated pure and mixed-component permeation analysis:
- We have partnered with Maxwell Robotics to develop automated pure and mixed-gas permeation systems, allowing for high-throughput materials characterization for both pure and mixed-gas permeation and diffusion properties. A comparison of the testing procedure between the automated and manual gas permeation systems is shown in the video below.
- Molecular diffusion and thermodynamic partitioning analysis:
- Maxwell Robotics has also developed automated high-pressure sorption systems, allowing for accurate thermodynamic partitioning and diffusion analysis. The lab is also equipped with a Brunauer-Emmett-Teller (BET) machine to analyze low temperature and pressure molecule diffusion and sorption.
- Materials stability to toxic gases:
- The lab has the capability to test pure and mixed-gas permeation of toxic and sour gases such as CO, SO2, and H2S. These gases are generally present in real-world natural gas purification separations and allow for testing of materials stability under realistic industrial conditions.